Decoding cell transport systems
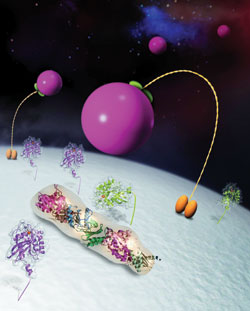
This fanciful world is actually an artistic rendering of the inside of a cell, showing TRAPP I lying on the Golgi (white "planet") and assisting in the tethering of vesicles (pink "moons").
Courtesy of Michael Sacher
For biologist Michael Sacher, “Everything has peaked at just the right time.” He is principal investigator on one of five Concordia projects to be awarded Canadian Institutes of Health Research (CIHR) funding this year.
CIHR made the news recently when it announced a significant decrease in the success rate of grant applicants. The drop is being attributed to an increase in the number of applicants and corresponding decrease in the total amount of funding available for research.
“We were informed early on that the success rate had dropped from what has been a fairly steady 25 per cent to around 15 per cent,” Sacher said. CIHR’s final figures put the national success rate at 16.4 per cent (see below).
Sacher’s application, “Identification and characterization of factors interacting with the multisubunit vesicle tethering complex TRAPP I,” was top-ranked in the field of Biochemistry and Molecular Biology (B). He will receive slightly over $118,000 per year over the next five years to further cell research that, to date, has involved colleagues at McGill, Harvard and Pohang University (South Korea).
As small as cells are, each one contains an almost complete world of structures and chemicals that regulate every bodily process. Microscopic compartments and even tinier amounts of proteins and lipids control everything from the timely release of insulin to how bones grow and heal.
Tiny vesicles (or sacs) carry proteins between different cellular compartments. “Each cell compartment contains a different set of proteins, we’re interested in knowing how those proteins get to the right compartments in a regular and timely fashion.”
“They best analogy I can offer is air travel,” he said, where each cell compartment is a city and the vesicles are planes. Not only do they always land at the right airport, they also get to the right arrival gate.
To figure out the mechanisms at work, Sacher and colleagues have been looking at vesicles that move between two cellular compartments, the endoplasmic reticulum (ER) and the Golgi apparatus.
Here the key appears to be TRAPP I, a large protein complex that acts as a vesicle tether and recognizes only those vesicles which belong. “To stretch the analogy, it’s the Golgi’s control tower. It only lets the right vesicles in.”
His research focuses on TRAPP I and its transport function at the Golgi apparatus as a model for understanding how other such vesicle tethers function.
To study proteins, you have to have access to them. As Sacher pointed out, “to get micrograms of a substance for laboratory use, there needs to be bucket loads of it [relatively speaking] in a cell.”
Because TRAPP I “is really not abundant” in the cell, one of the first hurdles was finding a method for producing the large complex in the required quantities.
“We knew of a group in Korea who was working on the same protein complex,” he said. When they pooled their knowledge, they were able to get the entire TRAPP I complex to express and spontaneously assemble in E. coli.
“Once we realized that we could produce the complex, we wanted to know what it looked like,” so they contacted a team at Harvard who had the equipment to help.
Using both low-resolution single particle electron microscopy and higher resolution X-ray crystallography, they were able to produce an image of TRAPP I, and its associated proteins. “To date, it is the best view that anyone has produced of a vesicle tethering complex,” Sacher said.
The combination of processes for protein production and imaging were unique, and were published in the Nov. 17 issue of the journal Cell.
“People in the field are pretty excited about the work,” Sacher said. “The complex we’re studying is implicated in skeletal disorders, but the research is fundamental.”
Any process that relies on a particular protein being in a particular place at a particular time can potentially be studied using the methods developed for looking at TRAPP I, thereby affecting research into literally hundreds of diseases and disorders, including diabetes.
With the CIHR funding, Sacher hopes to delve deeper into TRAPP I and its function. He will continue to work with collaborators (and co-authors) Thomas Walz (Harvard), Byung-Ha Oh (Korea) and Mirek Cygler (McGill).
“The next steps will be to try and understand how the protein complex localizes specifically to the Golgi membranes and to identify other proteins that interact with the complex to help it localize properly and whose function may be regulated by TRAPP I.”
“The Architecture of the Multisubunit TRAPP I Complex Suggests a Model for Vesicle Tethering,” by Yeon-Gil Kim, Stefan Raunser, Christine Munger, John Wagner, Young-Lan Song, Miroslaw Cygler, Thomas Walz, Byung-Ha Oh and Michael Sacher, can be found in Cell 2006 127.